However, access to suitable transplants, ethical aspects and a large variation in safety, quality and efficacy of donation and transplantation are constant and remaining issues. The unmet need for the receiving patient and the shortage of (suitable) transplants still leads to personal drama’s worldwide. According to the U.S. Government Information on Organ Donation and Transplantation, 109,000 men, women and children are on the transplant waiting list and every 9 minutes a new person is added to this list(1).
Imagine being able to grow a replacement heart or liver that could be used in transplantation. Imagine the lives that could be saved and all the happiness that could be brought to patients and their loved ones. This is the fundamental idea behind tissue engineering which itself is poised to revolutionize medical intervention in the coming years.
So in this article we’re going to take a look at what tissue engineering and 3D bioprinting are, and also look at the important multiple roles that gelatin can play at each stage in this exciting new field of medical research.
What is tissue engineering?
Tissue engineering is the overall term of combining cells and a carrier material, often called scaffold. Biological molecules or mechanical stimulation can also be used with the aim to create a supportive environment for the cells, enabling functional support and finally transplantation. In human tissues, the cells are supported by a complex extracellular matrix (ECM). Collagens constitute the primary structural element of the ECM and provide tensile strength, regulate cell adhesion, support chemotaxis, cell migration and direct tissue development. Cells thus actively interact with the ECM via various surface receptors and these interactions can be of high importance to maintain cell functions. Unsurprisingly, gelatin, as a collagen derived product, can play a major role in tissue engineering at various steps of the process.
To make a tissue engineered construct scientists usually go over 3 major steps:
1. Typically it all starts with the in vitro expansion culture of the cell of interest, be it stem cells, primary isolated cells or cell lines, often on conventional tissue culture plastics. Depending on the cell source, this step can have some challenges like troublesome adhesion and/or expansion of the (often primary) cells or even loss of function. In this phase, Rousselot X-pure® gelatins can be used to coat the tissue culture surface, providing cell recognition motives to the cells to prevent or diminish these types of problems. Furthermore, Rousselot X-pure® gelatins have low endotoxin levels (< 10 EU/g) such as LPS that can interfere with cell differentiation and expansion.
2. Once a sufficient number of cells is reached, the cells are combined with a carrier or scaffold. The material of the carrier is referred to as a biomaterial. These biomaterials are biocompatible and specially created for therapeutic or diagnostic purposes. The desired material characteristics highly depend on the envisioned end construct. Desired characteristics are bio-compatibility, cell-interactivity, tunable physico-chemical properties and biodegradability. Depending on the targeted end product, cells could be combined with the material by cell seeding on the carrier or by encapsulation in the material, mimicking the ECM environment of the cells.
As collagen derived products, gelatins serve as an excellent choice in this phase. They can play multiple roles :
- it could be used as coating to make another biomaterial carrier cell interactive and supportive. Rousselot X-pure® gelatins are an excellent choice for this purpose (see coating step for expansion)
- As a crosslinked hydrogel, it could serve as scaffold or carrier itself.
At Rousselot we provide X-pure® gelatins and modified gelatins that can be crosslinked to hydrogels for cell seeding. In case encapsulation is desired, we provide X-pure® GelMA, a purified (low levels of endotoxins and methacrylic acid) and modified gelatin that allows a biocompatible crosslinking enabling cell encapsulation. Our products have a high quality and reproducibility, and have highly tuneable physico-chemical properties and degradation rates making them an excellent choice as carrier material.
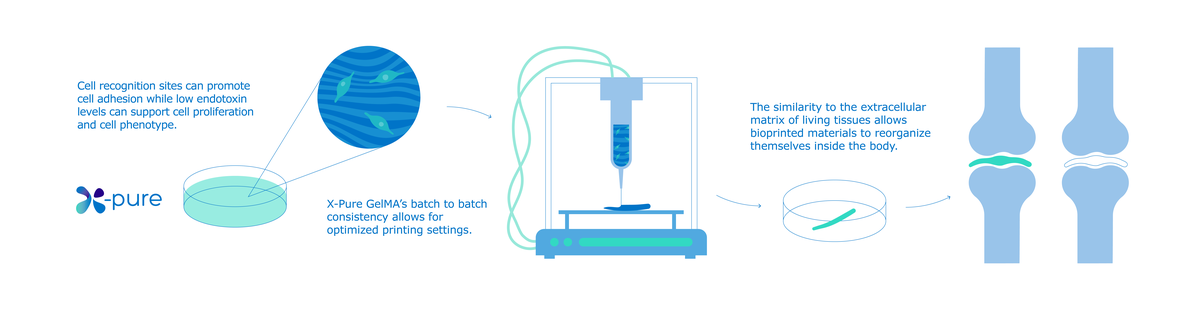
3. After this phase, the construct often undergoes a final cultivation step. This cultivation step can be done under static or dynamic conditions, depending on the envisioned end construct/tissue. One goal is to let cells grow and form a tissue, another goal is to enable differentiation of cells to exert their final function. This is often done by exposing the construct to mechanical and/or chemical stimulation. Be it under dynamic conditions or static. This final step also ensures the printed tissue has proper structural and functional integrity to survive transplantation.
In general, these steps in tissue engineering lead to a transplantable construct. Depending on the end-goal this ranges from making a simple construct, providing a transplantation carrier to one specific cell type, up to creating a complex fully functional tissue comprising multiple cell types trying to mimic the micro-architecture of the tissue. When an increased complexity and certain level of precision is desirable, tissue engineers often turn to 3D bioprinting, a very specialized part of tissue engineering.
A role for 3D Bioprinting in tissue engineering?
You’ve probably heard of ‘3D Printing’, the process of printing physical objects. So put simply, 3D Bioprinting is the process of printing biological parts such as tissue and organs. As with regular 3D printing, 3D bioprinting starts off with a computer generated blueprint that is printed layer by layer. The difference with 3D bioprinting, however, is that living cells and biomaterials (such as gelatin methacrylate - GelMa) are used instead of plastic.
Before start printing complex tissues, optimal bio-ink conditions are selected. Bio-ink is a combination of a biocompatible biomaterial and specific type(s) of cells, depending on the envisioned replacement tissue. The selection and creation of the bio-ink is a first crucial step and typically needs fine-tuning for different target cells/tissues The selected biomaterial should be biocompatible, possessing certain mechanic characteristics and allow the embedded cells to exchange nutrients and oxygen with their environment. For these reasons, hydrogels are particularly suitable for printing.
After a first selection of parameters, the bio-ink is loaded in the cartridges ready for printing. Based on the design parameters as defined in the computer software, the printer can generate the construct as determined by the original blueprint. Complexity can range from a simple one cartridge set-up comprising one type of bioink up to multiple cartridges comprising multiple cell types and/or biomaterial combinations. Typically, the printing process also requires some fine-tuning and feeds back to the bio-ink selection parameters to enable a bio-ink that is perfect from printing and cell biocompatibility perspective.
Thanks to its versatility and tunable physicochemical properties, gelatin allows the development of both high and low viscosity gelatin based bioinks. The gelling can be controlled by temperature, which facilitates optimization of the flow behavior during bioprinting. To create bioprinted hydrogels with a good mechanical stability at physiological temperatures under a controlled biodegradability process over time, a modified gelatin, gelatin methacryl anhydride (GelMA) was created. The GelMA can be crosslinked under controllable conditions by adding a crosslinking reagent. GelMA is probably the most used and studied modified gelatin for bioprinting. It has been shown ideal as bio-ink component because it reduces viscosity and gelling, both of which can impact negatively on the printed structures. In addition, it is chemically similar to the extracellular matrix that naturally gives structure to native tissue, meaning cells can easily enter it and reorganise themselves into a living structure. To conclude, GelMa is a versatile biomaterial that can be used in bioprinting to obtain biocompatible cell laden constructs with high structural resolution and shape enabling scientists to make the most of its unique properties.
To summarize, 3D bioprinting is an exciting new technology that has become an important part of tissue engineering. It is hoped that one day scientists will be able to print complex organs such as hearts and livers that could be used to save lives and improve the quality of life for patients suffering from serious diseases and injuries.
Why use Rousselot’s products for tissue engineering?
Despite their unique properties, the use of gelatins and GelMA can be challenged by the presence of impurities such as endotoxins (lipopolysaccharides) or residual methacrylic acid (MA). The presence of these impurities can negatively impact the quality of the research. When used in the body they can also lead to tissue inflammation, increased sensitivity to other allergens, and in extreme cases even fatal shock. Selecting purified biomaterials, such as X-Pure® gelatins and modified gelatins, specifically developed by Rousselot to overcome these challenges, is important.
Rousselot’s X-pure® gelatins and X-pure® GelMA have the following advantages:
- Biocompatibility. It’s unlikely to be rejected by the body.
- Low antigenicity. Purified gelatin is low in endotoxins, meaning it’s unlikely to cause an immune reaction.
- (Tunable) biodegradability. This means that gelatin can be naturally absorbed by the body.
- Tunable physicochemistry. Gelatin can be specially modified to suit a variety of applications.
- Cost-effectiveness. Gelatin is abundant meaning it can help tissue engineers to keep the costs down.
- A trusted biomaterial with a long track-record. Gelatin, the starting material for the Rousselot X-pure® range, has been used for over a century in various pharmaceutical applications such as capsules and medical applications such as hemostats and blood plasma substitutes.
- Meets high safety and compliance standards. This helps to ensure the overall safety of the engineered tissue.
Rousselot’s products enable scientists to make the most of gelatin’s unique properties and to successfully conduct reproducible, reliable and original scientific research ready to scale up for clinical trials.
(1) Organ Donation Statistics 2020, accessed on 17.12.2020 < https://www.organdonor.gov/statistics-stories/statistics.html